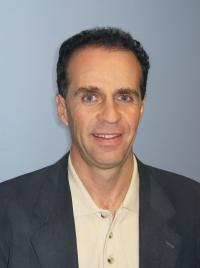
Alex Vitkin is an engineering physicist / biomedical engineer by training, with further specialization in medical physics and applications of lasers in medicine. He is currently a professor of Medical Biophysics and Radiation Oncology at the University of Toronto, and a medical physicist and Senior Scientist at Princess Margaret Cancer Centre, UHN.
His research is in the field of biophotonics, with particular emphasis on structural and functional optical coherence tomography, tissue polarimetry and optical fiber sensors. He has published >200 peer-reviewed papers and book chapters on diagnostic and therapeutic uses of light in biomedicine and holds several patents in the field; his laboratory works closely with clinicians and with industry, and he is a consultant for several biophotonics companies. He is a regular research grants panelist / site visitor / reviewer for NIH, CIHR, NSERC, CIMIT, ZonMw and many other national and international granting agencies.
Dr. Vitkin lectures widely, delivering special seminars and summer school modules on biophotonics in Brazil, China, Colombia, Cyprus, Finland, Germany, India, Ireland, Mexico, New Zealand, Russia, Taiwan, USA, Ukraine and Vietnam; he is currently an active participant in the SPIE Visiting Lecturer and OSA Travelling Lecturer programs. Dr. Vitkin is also a board-certified medical physicist through the Canadian College of Physicists in Medicine (CCPM) and is a Fellow of the Optical Society of America (OSA) and the Society of Photo-Optical Instrumentation Engineers (SPIE).